Circular RNAs: a novel tool in cardiovascular biomarker development?
Cardiovascular personalised medicine and biomarkers
As we move towards the era of cardiovascular personalised medicine (1), the development of novel and useful tools to support individualised patient care is assuming increasing importance (2). This new paradigm will provide a robust framework to inform diagnosis and prevention, and to guide therapy. A personalised approach will ultimately lead to improved clinical outcomes and increased quality of life for patients, as well as cost-effective benefits for healthcare systems. In this context, the identification of reliable biomarkers to assist in the decision-making process and risk assessment is a key step for translational and clinical research (3).
The National Institutes of Health working group defined a biomarker as a characteristic that is objectively measured and evaluated as an indicator of normal biological processes, pathological processes or pharmacological responses to therapeutic interventions (4). According to Morrow and de Lemos (5), novel biomarkers should fulfil a number of criteria to be useful in clinical practice. Namely, they should be measurable in routine practice with high accuracy, reproducibility and reasonable cost; should add new information that is not already available; and should assist the clinician to manage patients in decision-making and enhancing clinical care. This definition includes variables of a diverse nature. Nonetheless, the identification of non-invasive and easily accessible biomarkers should especially be considered in the cardiovascular field. Biopsy-based methods have limited applicability due to their invasiveness. Imaging methods increase the cost of medical care, require specialised centres and personnel, can jeopardize patient outcomes (exposure to radiation) and in some cases are impractical for large-scale population screening. The relatively simple withdrawal procedure for liquid biopsies suggests such biomarkers as the most intriguing method.
Previous examples have illustrated the successful development of circulating biomarkers in the cardiovascular arena. Cardiac troponin (cTnT) has emerged as a potent tool for the diagnosis of acute coronary syndrome (6). Nonetheless, recent data still casts some doubts as to whether circulating biomarkers support clinical decision-making (7). The Guiding Evidence Based Therapy Using Biomarker Intensified Treatment in Heart Failure (GUIDE-IT) study, a large prospective and multicentre clinical trial designed to compare the optimized guideline-recommended therapy and the N-terminal prohormone of brain natriuretic peptide (NT-proBNP)-guided therapy in high-risk patients with heart failure (HF), was prematurely terminated because of the lack of differences between treatment groups (8). Genetics has been explored in recent years. Although useful for evaluating disease susceptibility and providing mechanistic insights, the inherited genome provides an incomplete picture of the phenotype (9). Additional approaches are needed for the development of biomarkers in cardiovascular personalised medicine.
Non-coding transcriptome in biomarker development
The transcriptome represents all genes expressed in a cell in a given biological state. According to results from the Encyclopedia of DNA Elements (ENCODE) Project (10), it has been suggested that while 80% of the human genome is transcribed, only 1–3% represents protein-coding genes (11). Transcripts that do not encode proteins represent the vast majority of the human transcriptome (97–99%): non-coding RNAs (ncRNAs). ncRNAs play a significant role in a wide array of cellular processes and have been proposed as a main cause of biological complexity (12).
The ncRNA landscape provides vast opportunities for personalised medicine (9,13). The ncRNA signature may be indicative of the physiological and pathological status of the subject. The deregulation of the ncRNA profile is a hallmark of several human diseases, including cardiovascular disease (CVD) (14-16). Furthermore, the ncRNA patterns correlate with patient phenotype and may be informative of the interaction between the genotype and lifestyle-environmental factors, such as diet, physical activity, psychosocial stress or pollution (17-19). Therefore, ncRNAs may represent a novel source of information beyond traditional variables or genome biomarkers. Overall, the non-coding transcriptome has emerged as a new source of indicators for clinical practice (20). In 2012, the Food and Drug Administration (FDA) approved the long non-coding RNA (lncRNA) prostate cancer antigen 3 (PCA3), the Progensa® PCA3 assay, as a urine-based molecular diagnostic test. PCA3 is a lncRNA that is highly overexpressed in prostate cancer and can be detected in urine (21). Different meta-analyses have demonstrated that urine PCA3 can be used to guide diagnosis with an acceptable sensitivity and specificity (22,23).
A search of the scientific literature clearly indicates that circulating microRNAs (miRNAs) and lncRNAs are the main classes of ncRNAs that have been studied as biomarkers of CVD (24-27). Recent advances in RNA sequencing (RNA-Seq) and bioinformatic analysis have drawn attention to another promising member of the ncRNA family: circular RNAs (circRNAs). Although previous publications have addressed the potential of circRNAs as cardiovascular biomarkers, this research field has not been revised in detail. Here, we intend to summarize current knowledge of circRNAs as biomarkers for the diagnosis and prognostication of cardiovascular conditions.
circRNAs
CircRNAs are single-stranded, covalently closed RNA molecules that lack free caps or poly(A) tails. These circRNAs are generated by back-splicing, which links a downstream 5' splice site to an upstream 3' splice site, forming a closed, continuous loop. The biogenesis of circRNAs is not fully understood [for more information about circRNA biogenesis: (28)], but the circRNAs represent a heterogeneous group of transcripts that are mainly formed by exons and can also be derived from intronic, non-coding, antisense, untranslated or intergenic genomic regions (29). Their size ranges from hundreds to thousands of nucleotides. Many putative circRNAs with distinct expression profiles can be produced from a single gene (30). Although circRNAs were previously described as rare compared to linear RNA, various studies have demonstrated that circular isoforms can be the predominant transcript isoform for a number of genes (30). Furthermore, their expression is not necessarily correlated with the expression of the linear transcripts from which the circRNA is derived, suggesting a complex regulatory mechanism (31). Although they are predominantly cytoplasmic (29), their presence has also been described in the nucleus (32). CircRNA expression appear to be associated with the proliferative status of cells, and their levels are higher in differentiated cells, such as in the brain (33).
Circular transcripts were first described in the 1970s. Nonetheless, they were initially considered artefacts or non-functional, accidental transcription by-products. Recently, RNA-Seq data identified and characterised the ubiquitously expression of thousands of circRNAs in human cells (34). The circRNAs are expressed in many species from yeast to humans, and are highly conserved in eukaryotes (31,34), pointing to a key role in relevant biological processes. Evidence of possible functions is continuously emerging. circRNAs harbour miRNA binding sites (35) and can act as miRNA sponges in competing endogenous RNA (ceRNA) networks (36), thus affecting gene expression. Strikingly, they can also stabilize miRNAs and serve as miRNA reservoirs (37). CircRNAs regulate RNA transcription by binding to RNA polymerase II (32) or DNA (38), and can interact with RBPs to regulate their function (39). Although still controversial, these circular transcripts could function as coding transcripts (40).
The involvement of circRNAs at the onset and development of many conditions has been proposed, including CVD [reviewed in (41,42)]. Thus, circRNAs have emerged as promising therapeutic targets and biomarkers of disease.
circRNAs as novel biomarkers with clinical application
The circRNAs possess various chemical and biological properties that are useful to become a new entity of clinical indicators. The circRNAs are highly stable, due to the lack of 3’ and 5’ terminal ends that are exposed to the degradation by exoribonucleases and certain RNA folding (34,43). Indeed, cellular circRNAs exhibit half-lives longer than 48 h, which are higher than those for linear RNAs (34).
There are a plethora of examples in the literature that suggest the cell- and developmental stage- and disease-specific expression of circRNAs. Maass et al. (44) identified 2,339 unique circRNAs in platelets when comparing 20 human cell types and tissues. These results were corroborated by an independent study that demonstrated that the most abundant circRNA in platelets, Plt-circR4, was exclusively expressed in platelets when comparing 10 different cell lines (45). The proportion of circRNAs in megakaryocytes differs from that observed in platelets (46). The results in alternative cell-types support cell and developmental specificity. Indeed, 939, 333 and 194 circRNAs were exclusively expressed in CD19+ cells, CD34+ cells and neutrophils, respectively (29). Specific circular isoforms from the same gene have been observed in various tissues (44). The expression profiles of circRNA show specific changes in pathological conditions and have been linked to cellular processes such as proliferation, migration and cell death (47,48). Interestingly, a transient middle cerebral artery occlusion in mice induced a specific pattern of circRNA deregulation in the penumbral cortex at 6, 12, and 24 h of reperfusion (49) which suggests the spatiotemporal expression of circRNAs in pathological conditions. Furthermore, unique circRNAs, such as fusion-circRNAs (f-circRNAs) derived from cancer-associated chromosomal translocation, have been detected in leukaemia (50). These circRNAs represent an interesting diagnostic tool for clinical practice. Notably, circRNAs are relatively well-conserved in a broad range of species, which facilitates investigation in various cellular and animal models.
The circRNAs are easily detected in clinical standard samples using relatively accessible methodologies: RNA-Seq, microarray, and reverse transcription droplet digital polymerase chain reaction (RT-ddPCR), some of which are used in clinical laboratories, such as reverse transcription real-time quantitative PCR (RT-qPCR) (51-54). Using a RNA-Seq approach in two biological replicates, Memczak et al. (55) identified more than 4,000 unique circRNA candidates in clinical whole blood specimens, with approximately 2,400 circRNAs reproducibly detected. The circRNAs have also been described in platelets, leukocytes and red blood cells (44,46). The circRNA signature of blood components could be used in biomarker-based studies. Although the exact number of circRNAs in plasma and serum samples is still controversial, various studies have described the presence of cell-free circRNAs in the circulation. Sequencing of ribosomal RNA-depleted total RNA demonstrated the expression of 57 and 39 circRNAs in plasma and serum, respectively (44). A microarray analysis demonstrated the presence of a mean number of 18,293 circRNAs (range, 16,185–18,954) in 21 plasma samples from eight cancer patients (56). The detection of thousands of circRNAs in the cell-free compartment constitutes an advantage in terms of information provided compared to the main ncRNAs evaluated in biomarker-based studies, miRNAs, hundreds of which can be reliably detected in plasma/serum samples (57). The circRNAs have also been detected in other clinically relevant specimen, such as saliva (58) and gastric juice (59). Additionally, extracellular vesicles could also be used as a reservoir of circRNA-based biomarkers. Cellular export via extracellular vesicles may be a common property of many cell types, as demonstrated by different studies that have detected circRNAs in exosomes and/or microvesicles released from different cell types (60-62). Li et al. (63) identified 1,215 circRNAs in human exosomes isolated from serum obtained from healthy donors. Authors reported that the incubation of serum at room temperature for up to 24 h had a minimal effect on circRNA expression levels, which provides a clue about the possible stability of extracellular circRNAs. Additionally, the same study described the presence of human circRNAs in the serum of a xenograft mouse model of MHCC-LM3 cancer cells. In particular, human circRNA CDYL correlated with tumour mass, suggesting the potential of circRNAs as biomarkers of disease.
Similar to their intracellular counterparts, extracellular circRNAs can be modulated by various physiological and pathological states. For example, a temporal dynamic regulation of circulating circRNAs has been observed for the three trimesters of pregnancy (52). A number of studies have suggested that extracellular circRNA levels in clinical specimens are biomarkers of diverse pathological conditions (54,60,64-78), especially in cancer (51,53,56,63,79-87)(summarized in Figure 1). Interestingly, Broadbent et al. (88) reported the expression of protozoan circRNAs during the blood stages in the development of malaria. Therefore, exogenous circRNAs should also be considered in biomarker development.
circRNAs as biomarkers of CVD
The use of novel ‘omic’ approaches have allowed the identification of thousands of circRNAs in cardiovascular cells and tissues from humans and different animal models (89-91). Specific circRNAs have been reported to be deregulated in pathological conditions such as ischaemic disease, HF and cardiomyopathy (89,92-94). It could be hypothesised that in conditions of cellular stress or injury, circRNAs are actively secreted in extracellular vesicles or passively released upon cell necrosis (Figure 2), and thus, their circulating profile may be indicative of pathological processes in the cardiovascular system. Nevertheless, since CVD has a complex etiopathology that involves a great number of cells and tissues, circRNAs of a non-cardiovascular origin should also be considered. For example, circRNAs have been implicated in the regulation of inflammatory responses and the binding of leukocytes to endothelium cells (95). Although still in its infancy, previous results in this area suggest the potential of circRNAs as biomarkers of cardiovascular conditions (Figure 1). In this section, we will review publications that have proposed circRNAs as diagnostic and prognostic cardiovascular biomarkers.
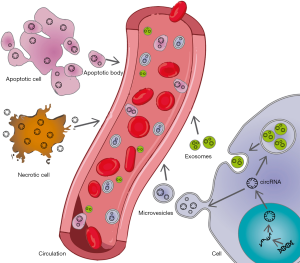
circRNAs and atherosclerotic-related disease
Coronary artery disease (CAD) is the leading cause of death worldwide. The identification of novel biomarkers of atherosclerotic-related conditions is a hot topic in cardiovascular research (96). In this context, ncRNAs have been extensively investigated as biomarkers of atherosclerotic-related conditions over the last decade (97,98). Zhao et al. (76) evaluated the potential of peripheral blood circRNAs as biomarkers of CAD. Using a microarray approach, they first observed 22 circRNAs that were differentially expressed between 12 CAD patients and 12 matched control subjects. Then, the 5 circRNAs with the highest fold changes were validated in a cohort of 30 control subjects and 30 patients with different CAD severity using RT-qPCR. One of these deregulated circRNAs, hsa_circ_0124644, showed a high discrimination ability [AUC (95% CI) =0.872 (0.785–0.960)]. The results were confirmed in an independent cohort consisting of 137 CAD patients and 115 control subjects [AUC (95% CI) =0.769 (0.710–0.827)]. The blood levels of the circRNA hsa_circ_0082081, hsa_circ_0113854 hsa_circ_0124644 and hsa-circRNA5974-1 were correlated with the severity of CAD, pointing to a possible role of circRNAs as biomarkers of the disease stage. Using a multimarker approach, authors demonstrated that the combination of the circRNA hsa_circ_0124644 and other candidate, hsa_circ_0098964, improved the diagnostic value [AUC (95% CI) =0.811 (0.756–0.865)]. Supporting a causal relationship, but did not demonstrate, the results of gene ontology enrichment suggested the participation of hsa_circ_0124644 in atherosclerosis progression through the regulation of various cellular processes, including apoptosis and intercellular adhesion. In a similar study, Pan et al. (69) evaluated plasma ceRNA expression profiles to identify novel diagnostic biomarkers of CAD. Again, based on a microarray approach, the authors identify 24 circRNAs that are differentially expressed, 18 that are upregulated and 6 that are downregulated, among three pairs of plasma samples from control subjects and CAD patients. Nine of these circRNAs, hsa_circ_0006323, hsa_circ_0032970, hsa_circ_0051172, hsa_circ_0054537, hsa_circ_0057576, hsa_circ_0068942, hsa_circ_0082824, hsa_circ_0083357 and hsa_circ_0089378, have binding sites for one miRNA, miR-130a-3p, that regulated the mRNA transient receptor potential cation channel subfamily M member 3 (TRPM3). Of note, miR-130a-3p was previously associated with coronary atherosclerosis (99). Although the in vivo relevance of these findings should be explored, the results suggested that the identification of regulatory networks including circRNAs could be a novel source of biomarkers and therapeutic targets.
The circRNAs appear to be biomarkers themselves as well as in combination with other ncRNAs. CircR-284 is a circRNA expressed in human vascular smooth muscle cells (VSMCs) and carotid plaques that have a binding site for miR-221 (60). Remarkably, the serum ratio of circulating circR-284 to miR-221 has been demonstrated to be elevated in patients with urgent ischemic cerebrovascular event within the previous 5 days (N=17) compared to asymptomatic patients (N=24) (60). Furthermore, this ratio was an excellent diagnostic test for carotid plaque rupture leading to an acute ischaemic event in a validation study with 112 patients [AUC (95% CI) =0.98 (0.96–1.00), sensitivity =93.0%, specificity =97.0%]. The relative levels of circR-284 to miR-221 were not associated with clinical variables, including age, sex, BMI, smoking, or different medications, which boosts its possible clinical application as a biomarker. Whether this ratio has any biological relevance deserves further investigation.
circRNAs and hypertension
Hypertension is a complex and multifactorial disease that constitutes one of the main risk factors for CVD. Some studies have previously evaluated the association between the circulating circRNA signature and this condition. Using a microarray analysis, Wu et al. (73) explored the expression profile of circRNAs in the plasma of 5 hypertensive patients and 5 healthy controls. Fifty-nine circRNAs were identified as differentially expressed in hypertensive patients. Based on the fold-change and P value, 4 circRNAs (hsa-circ-0000437, hsa-circ-0008139, hsa-circ-0005870 and hsa-circ-0040809) were selected for further validation in a cohort of 49 hypertensive patients and 49 healthy individuals using RT-qPCR. Consistent with microarray findings, the plasma levels of hsa-circ-0005870 were downregulated in hypertensive patients. Authors established the predicted hsa-circ-0005870 targeted circRNA-miRNA-mRNA network based on sequence-pairing prediction. The results yielded a network of a total of 5 miRNAs and 849 mRNAs that were predicted to interact with this circRNA. KEGG (Kyoto Encyclopedia of Genes and Genomes) pathway analysis pointed to a role in the TGF-beta signalling pathway, a key pathological mechanism in hypertension (100). In an additional study, Bao et al. (64) evaluated the circRNA signature in blood samples from patients with essential hypertension (EH). Following microarray analysis, they reported that hsa_circ_0037911 was differentially expressed in a case-control study of 100 EH patients and 100 age- and gender-matched healthy subjects. In logistic regression models, hsa_circ_0037911 demonstrated a higher association with EH than other established clinical variables. Nonetheless, additional analyses are needed to elucidate whether is a biomarker of hypertension. Indeed, the discrimination ability is poor (AUC =0.627) compared to other circRNA-based studies and has_circ_0037911 showed differences according to gender, smoking and alcohol drinking among EH patients.
circRNAs and aneurysm
Intracranial aneurysm (IA) is a serious neurological condition with poor prognosis and high mortality rates (101). At present, the management of IA is limited by the clinical tools available for diagnosis and prognosis. Therefore, there is great interest in the development of new biomarkers that could allow the identification of patients at high risk for adverse outcomes. A recent study has evaluated the potential of a circRNA, hsa_circ_0021001, as a diagnostic and prognostic biomarker of IA in a cohort of 223 cases of IA patients and 131 healthy volunteers (71). In this case, the selection of hsa_circ_0021001 was based on the circ2Traits database (http://gyanxet-beta.com/circdb/), which predicted an association between circRNA and IA. Using a RT-qPCR approach in peripheral blood samples, the authors observed that hsa_circ_0021001 expression in IA patients was significantly lower compared to healthy controls and that this circRNA showed good discrimination ability [AUC (95% CI) =0.87 (0.784–0.963), sensitivity =81.0%, specificity =92.0%]. Cox regression models demonstrated that hsa_circ_0021001 expression, together with other established clinical variables, was a prognostic risk factor for IA. Interestingly, hsa_circ_0021001 expression was independent of a number of risk factors, including age, gender, size of aneurysm, hypertension history, smoking, alcohol consumption, coronary heart disease, blood glucose, and aneurysm location, which suggests that this circRNA can provide additional information over established clinical variables.
circRNAs and cardiovascular outcomes
In recent years, various studies have explored the circulating circRNA signature as a tool to identify patients at risk of cardiovascular adverse events. The findings point to the circRNAs as novel indicators to accurately identify patients at risk of adverse outcomes. Vausort et al. (72) explored the prognostic potential of the circRNA that was designated the myocardial infarction associated circular RNA (MICRA) as a predictor of LV dysfunction after 3 to 4 months of acute myocardial infarction (MI) in whole blood samples from two independent cohorts (total sample size =642). First, the authors demonstrated that peripheral blood levels of MICRA were lower in MI patients than in healthy controls. Then, multivariate logistic regression analysis revealed that lower levels of MICRA were associated with a higher risk of LV dysfunction [OR (95% CI) =0.10 (0.04–0.25) for univariate model, OR (95% CI) =0.08 (0.03–0.24) for multivariate model]. Furthermore, MICRA provided an incremental value to predict HF development over established clinical variables and biomarkers, including age, sex, cardiovascular risk factors, cTnT, NT-proBNP and CPK, among others [IDI (95% CI) =0.07 (0.02–0.12)]. These findings were corroborated in a validation cohort and replicated in a later study (N=472) using an alternative risk stratification system (70). Interestingly, the authors hypothesised a possible sponge effect of miR-150, a miRNA previously reported as a biomarker of LV remodelling after MI by the same group (102).
Zhang et al. (74) recently revealed that the plasma levels of hsa_circRNA_025016 before surgery predicted new-onset atrial fibrillation (AF) after isolated off-pump coronary artery bypass grafting. First, a microarray approach was used with plasma samples collected 1 week before surgery for 15 pairs of patients with new-onset AF and patients who did not develop AF matched for age, sex, smoking, left atrial diameter, end-diastolic volume, and statin use. The authors selected 9 candidates. After further testing via RT-qPCR, two circRNAs, hsa_circRNA_025016 [OR (95% CI) =1.355 (1.250–1.468)] and hsa_circRNA_404686 [OR (95% CI) =1.098 (1.009–1.195)], were stably detected in plasma and were differentially expressed between study groups and predicted AF (N=365). The hsa_circRNA_025016 showed good predictive performance in a training cohort of 365 patients [AUC (95% CI) =0.802 (0.777–0.811), sensitivity =79.4%, specificity =77.6%]. The results were corroborated in a validation cohort of 284 patients. The hsa_circRNA_025016 was also upregulated in patients with coronary heart disease compared with the healthy controls. Pathway analysis predicted possible participation in insulin secretion pathway. Although the results from this observational study do not support a causal relationship in this pathway, a positive correlation between circRNA plasma levels and fasting blood glucose was reported in both cohorts.
Limitations and perspectives
Similar to other members of the ncRNA family, it is anticipated that there would be a low reproducibility and a large discrepancy between groups. This situation could not be linked to the poor performance of ncRNAs as a biomarker, but novel technological platforms and additional efforts are needed for the standardization of the methodology and process automation.
Multicentre studies with higher geographical dispersion and larger populations are fundamental to validate previous findings. A detailed biomarker study should be performed to explore sensitivity and specificity. The results should be confirmed and validated in a number of specimens and independent cohorts. Circulating levels of circRNAs may be affected by multiple factors. Therefore, adequate control of confounding variables, including age, sex, cardiovascular risk factors, medication, disease stage and others, is needed. In addition, comparison with current diagnostic and prognostic biomarkers is a key step to evaluate the real potential of circRNAs in clinical practice, in terms of biomarker performance and cost-effectiveness. In this sense, most studies have been focused on a single circRNA. It may be interesting to explore single circRNAs, or a signature of circRNAs, in conjunction with clinical and physical assessment and established biomarkers, to provide better characterisation of the disease or outcome of interest. Recent advances in systems biology could be a useful tool for this purpose. The evaluation of circRNAs as tools to predict and monitor therapy should also be performed to fully evaluate their potential in cardiovascular personalised medicine.
The assessment of RNA in bodily fluids has strong methodological limitations, mainly due to their low concentration. Furthermore, it is still unclear which is the real half-life in extracellular fluids (103). RNA-Seq is the reference method in circRNA discovery. Nonetheless, this technique has relevant limitations: detection efficiency, cost, sample volume, RNA integrity and the complex interpretation of the data. There are also great differences among the algorithms proposed in the literature (104). The circRNA microarrays could be an interesting alternative (56). Contrary to RNA-Seq, microarrays can only detect known circRNAs. RT-qPCR is primary methodology used in large population studies. However, there is poor agreement between RNA-Seq- and microarray-based technologies and this technique (105). The implementation of more accurate methodologies, such as RT-ddPCR, appears to be fundamental.
The high variability among groups in the isolation procedures, detection-platforms and data analysis limits the replicability of results across different laboratories. Several studies have used different normalisation approaches, some with serious limitations for circulating ncRNAs analysis: GAPDH (71) and 18S (74). Because circRNAs are expressed in blood cells, haemolysis should be considered in the analysis of expression levels. There is a strong variability in the nomenclature used. The genomic position should be provided in the publications. In addition, the use of a reference database, similar to with miRBase (http://www.mirbase.org/) in the case of miRNAs, will be a necessary step in the future.
Different questions related to the biology of extracellular circRNAs must still be addressed. A causal link between extracellular circRNAs and the underlying CVD is currently lacking. Indeed, this situation is still unresolved for other members of the ncRNA family. Nonetheless, although recommended, biomarkers need not contribute to the disease mechanism to have utility. Candidates may function as mediators of disease, bystanders that are not associated with the disease, or integrators of multiple sources of information that are linked to the disease. Future studies should investigate the cellular source, its metabolism in the extracellular space and the modulation of recipient cell behaviours in addition to mechanisms that mediate circRNA production and secretion. Furthermore, whether the release is cardiovascular-specific or other tissues and organs are also implicated deserves further attention. It could be interesting to study the development of a circRNA tissue map, similar to those generated for miRNAs (106). Whether changes in extracellular circRNAs reflect corresponding changes in the cells or tissues of interest should also be evaluated. A low correlation has been demonstrated for other members of the ncRNA family (99). Finally, the circRNA presence in extracellular vesicles could be a protective mechanism that, together with their stability, precludes degradation by RNases in extracellular fluids. Nonetheless, the association of extracellular circRNAs with RNA-binding proteins or lipoproteins has not been evaluated.
Conclusions
The evidence presented here suggests the tremendous potential of circRNAs in cardiovascular biomarker research. However, their performance as clinical indicators is just beginning to be elucidated.
Acknowledgments
We thank the editors for inviting us to write this review. The authors apologize for not including all relevant articles in the field. DdG-C and VLl-C are members of the CardiolincTM network.
Funding: D de Gonzalo-Calvo was a recipient of a Juan de la Cierva-Incorporación grant from the Ministerio de Economía y Competitividad (IJCI-2016-29393). CIBER Cardiovascular (CB16/11/00403 to D de Gonzalo-Calvo and V Llorente-Cortes) is a project of the Instituto de Salud Carlos III.
Footnote
Conflicts of Interest: All authors have completed the ICMJE uniform disclosure form (available at http://dx.doi.org/10.21037/ncri.2018.06.06). The authors have no conflicts of interest to declare.
Ethical Statement: The authors are accountable for all aspects of the work in ensuring that questions related to the accuracy or integrity of any part of the work are appropriately investigated and resolved.
Open Access Statement: This is an Open Access article distributed in accordance with the Creative Commons Attribution-NonCommercial-NoDerivs 4.0 International License (CC BY-NC-ND 4.0), which permits the non-commercial replication and distribution of the article with the strict proviso that no changes or edits are made and the original work is properly cited (including links to both the formal publication through the relevant DOI and the license). See: https://creativecommons.org/licenses/by-nc-nd/4.0/.
References
- van der Leeuw J, Ridker PM, van der Graaf Y, et al. Personalized cardiovascular disease prevention by applying individualized prediction of treatment effects. Eur Heart J 2014;35:837-43. [Crossref] [PubMed]
- Lyman GH, Moses HL. Biomarker Tests for Molecularly Targeted Therapies--The Key to Unlocking Precision Medicine. N Engl J Med 2016;375:4-6. [Crossref] [PubMed]
- Libby P, King K. Biomarkers: A Challenging Conundrum in Cardiovascular Disease. Arterioscler Thromb Vasc Biol 2015;35:2491-5. [Crossref] [PubMed]
- Biomarkers Definitions Working Group. Biomarkers and surrogate endpoints: preferred definitions and conceptual framework. Clin Pharmacol Ther 2001;69:89-95. [Crossref] [PubMed]
- Morrow DA, de Lemos JA. Benchmarks for the assessment of novel cardiovascular biomarkers. Circulation 2007;115:949-52. [Crossref] [PubMed]
- Thygesen K, Alpert JS, Jaffe AS, et al. Third universal definition of myocardial infarction. Eur Heart J 2012;33:2551-67. [Crossref] [PubMed]
- de Gonzalo-Calvo D, Davalos A, Fernandez-Sanjurjo M, et al. Circulating microRNAs as emerging cardiac biomarkers responsive to acute exercise. Int J Cardiol 2018;264:130-6. [Crossref] [PubMed]
- Felker GM, Anstrom KJ, Adams KF, et al. Effect of Natriuretic Peptide-Guided Therapy on Hospitalization or Cardiovascular Mortality in High-Risk Patients With Heart Failure and Reduced Ejection Fraction: A Randomized Clinical Trial. JAMA 2017;318:713-20. [Crossref] [PubMed]
- Costantino S, Libby P, Kishore R, et al. Epigenetics and precision medicine in cardiovascular patients: from basic concepts to the clinical arena. Eur Heart J 2017; [Epub ahead of print]. [Crossref] [PubMed]
- ENCODE Project Consortium. An integrated encyclopedia of DNA elements in the human genome. Nature 2012;489:57-74. [Crossref] [PubMed]
- Pennisi E. Genomics. ENCODE project writes eulogy for junk DNA. Science 2012;337:1159, 1161.
- Liu G, Mattick JS, Taft RJ. A meta-analysis of the genomic and transcriptomic composition of complex life. Cell Cycle 2013;12:2061-72. [Crossref] [PubMed]
- de Gonzalo-Calvo D, Kenneweg F, Bang C, et al. Circulating Long Noncoding RNAs in Personalized Medicine: Response to Pioglitazone Therapy in Type 2 Diabetes. J Am Coll Cardiol 2016;68:2914-6. [Crossref] [PubMed]
- Barwari T, Joshi A, Mayr M. MicroRNAs in Cardiovascular Disease. J Am Coll Cardiol 2016;68:2577-84. [Crossref] [PubMed]
- Uchida S, Dimmeler S. Long Noncoding RNAs in Cardiovascular Diseases. Circ Res 2015;116:737-50. [Crossref] [PubMed]
- Thum T, Condorelli G. Long Noncoding RNAs and MicroRNAs in Cardiovascular Pathophysiology. Circ Res 2015;116:751-62. [Crossref] [PubMed]
- de Gonzalo-Calvo D, Dávalos A, Montero A, et al. Circulating inflammatory miRNA signature in response to different doses of aerobic exercise. J Appl Physiol 1985;2015:124-34. [PubMed]
- Gil-Zamorano J, Martin R, Daimiel L, et al. Docosahexaenoic acid modulates the enterocyte Caco-2 cell expression of microRNAs involved in lipid metabolism. J Nutr 2014;144:575-85. [Crossref] [PubMed]
- Krauskopf J, de Kok TM, Hebels DG, et al. MicroRNA profile for health risk assessment: Environmental exposure to persistent organic pollutants strongly affects the human blood microRNA machinery. Sci Rep 2017;7:9262. [Crossref] [PubMed]
- de Gonzalo-Calvo D, Vea A, Bär C, et al. Circulating non-coding RNAs in biomarker-guided cardiovascular therapy: a novel tool for personalized medicine? Eur Heart J 2018; [Epub ahead of print]. [Crossref] [PubMed]
- Lee GL, Dobi A, Srivastava S. Prostate cancer: diagnostic performance of the PCA3 urine test. Nat Rev Urol 2011;8:123-24. [Crossref] [PubMed]
- Hu B, Yang H, Yang H. Diagnostic value of urine prostate cancer antigen 3 test using a cutoff value of 35 mug/L in patients with prostate cancer. Tumour Biol 2014;35:8573-80. [Crossref] [PubMed]
- Xue WJ, Ying XL, Jiang JH, et al. Prostate cancer antigen 3 as a biomarker in the urine for prostate cancer diagnosis: a meta-analysis. J Cancer Res Ther 2014;10:C218-21. [Crossref] [PubMed]
- Viereck J, Thum T. Circulating Noncoding RNAs as Biomarkers of Cardiovascular Disease and Injury. Circ Res 2017;120:381-99. [Crossref] [PubMed]
- de Gonzalo-Calvo D, Iglesias-Gutierrez E, Llorente-Cortes V. Epigenetic Biomarkers and Cardiovascular Disease: Circulating MicroRNAs. Rev Esp Cardiol (Engl Ed) 2017;70:763-9. [Crossref] [PubMed]
- Navickas R, Gal D, Laucevicius A, et al. Identifying circulating microRNAs as biomarkers of cardiovascular disease: a systematic review. Cardiovasc Res 2016;111:322-37. [Crossref] [PubMed]
- Bär C, Chatterjee S, Thum T. Long Noncoding RNAs in Cardiovascular Pathology, Diagnosis, and Therapy. Circulation 2016;134:1484-99. [Crossref] [PubMed]
- Chen LL. The biogenesis and emerging roles of circular RNAs. Nat Rev Mol Cell Biol 2016;17:205-11. [Crossref] [PubMed]
- Memczak S, Jens M, Elefsinioti A, et al. Circular RNAs are a large class of animal RNAs with regulatory potency. Nature 2013;495:333-8. [Crossref] [PubMed]
- Salzman J, Gawad C, Wang PL, et al. Circular RNAs are the predominant transcript isoform from hundreds of human genes in diverse cell types. PLoS One 2012;7:e30733 [Crossref] [PubMed]
- Salzman J, Chen RE, Olsen MN, et al. Cell-type specific features of circular RNA expression. PLoS Genet 2013;9:e1003777 [Crossref] [PubMed]
- Li Z, Huang C, Bao C, et al. Exon-intron circular RNAs regulate transcription in the nucleus. Nat Struct Mol Biol 2015;22:256-64. [Crossref] [PubMed]
- Rybak-Wolf A, Stottmeister C, Glazar P, et al. Circular RNAs in the Mammalian Brain Are Highly Abundant, Conserved, and Dynamically Expressed. Mol Cell 2015;58:870-85. [Crossref] [PubMed]
- Jeck WR, Sorrentino JA, Wang K, et al. Circular RNAs are abundant, conserved, and associated with ALU repeats. RNA 2013;19:141-57. [Crossref] [PubMed]
- Hansen TB, Jensen TI, Clausen BH, et al. Natural RNA circles function as efficient microRNA sponges. Nature 2013;495:384-8. [Crossref] [PubMed]
- Wang K, Long B, Liu F, et al. A circular RNA protects the heart from pathological hypertrophy and heart failure by targeting miR-223. Eur Heart J 2016;37:2602-11. [Crossref] [PubMed]
- Wang K, Sun Y, Tao W, et al. Androgen receptor(AR) promotes clear cell renal cell carcinoma(ccRCC) migration and invasion via altering the circHIAT1/miR-195-5p/29a-3p/29c-3p/CDC42 signals. Cancer Lett 2017;394:1-12. [Crossref] [PubMed]
- Conn VM, Hugouvieux V, Nayak A, et al. A circRNA from SEPALLATA3 regulates splicing of its cognate mRNA through R-loop formation. Nat Plants 2017;3:17053. [Crossref] [PubMed]
- Du WW, Yang W, Liu E, et al. Foxo3 circular RNA retards cell cycle progression via forming ternary complexes with p21 and CDK2. Nucleic Acids Res 2016;44:2846-58. [Crossref] [PubMed]
- Yang Y, Fan X, Mao M, et al. Extensive translation of circular RNAs driven by N(6)-methyladenosine. Cell Res 2017;27:626-41. [Crossref] [PubMed]
- Devaux Y, Creemers EE, Boon RA, et al. Circular RNAs in heart failure. Eur J Heart Fail 2017;19:701-9. [Crossref] [PubMed]
- Gomes CP, Salgado-Somoza A, Creemers EE, et al. Circular RNAs in the cardiovascular system. Non-coding RNA Research 2018;3:1-11. [Crossref]
- Beermann J, Piccoli MT, Viereck J, et al. Non-coding RNAs in Development and Disease: Background, Mechanisms, and Therapeutic Approaches. Physiol Rev 2016;96:1297-325. [Crossref] [PubMed]
- Maass PG, Glazar P, Memczak S, et al. A map of human circular RNAs in clinically relevant tissues. J Mol Med (Berl) 2017;95:1179-89. [Crossref] [PubMed]
- Preußer C, Hung LH, Schneider T, et al. Selective release of circRNAs in platelet-derived extracellular vesicles. J Extracell Vesicles 2018;7:1424473 [Crossref] [PubMed]
- Alhasan AA, Izuogu OG, Al-Balool HH, et al. Circular RNA enrichment in platelets is a signature of transcriptome degradation. Blood 2016;127:e1-e11. [Crossref] [PubMed]
- Kristensen LS, Hansen TB, Veno MT, et al. Circular RNAs in cancer: opportunities and challenges in the field. Oncogene 2018;37:555-65. [Crossref] [PubMed]
- Xu Z, Yan Y, Zeng S, et al. Circular RNAs: clinical relevance in cancer. Oncotarget 2017;9:1444-60. [PubMed]
- Mehta SL, Pandi G, Vemuganti R. Circular RNA Expression Profiles Alter Significantly in Mouse Brain After Transient Focal Ischemia. Stroke 2017;48:2541-8. [Crossref] [PubMed]
- Guarnerio J, Bezzi M, Jeong JC, et al. Oncogenic Role of Fusion-circRNAs Derived from Cancer-Associated Chromosomal Translocations. Cell 2016;165:289-302. [Crossref] [PubMed]
- Ji W, Qiu C, Wang M, et al. Hsa_circ_0001649: A circular RNA and potential novel biomarker for colorectal cancer. Biochem Biophys Res Commun 2018;497:122-6. [Crossref] [PubMed]
- Koh W, Pan W, Gawad C, et al. Noninvasive in vivo monitoring of tissue-specific global gene expression in humans. Proc Natl Acad Sci U S A. 2014;111:7361-6. [Crossref] [PubMed]
- Li T, Shao Y, Fu L, et al. Plasma circular RNA profiling of patients with gastric cancer and their droplet digital RT-PCR detection. J Mol Med (Berl) 2018;96:85-96. [Crossref] [PubMed]
- Zhao Z, Li X, Jian D, et al. Hsa_circ_0054633 in peripheral blood can be used as a diagnostic biomarker of pre-diabetes and type 2 diabetes mellitus. Acta Diabetol 2017;54:237-45. [Crossref] [PubMed]
- Memczak S, Papavasileiou P, Peters O, et al. Identification and Characterization of Circular RNAs As a New Class of Putative Biomarkers in Human Blood. PLoS One 2015;10:e0141214 [Crossref] [PubMed]
- Li S, Teng S, Xu J, et al. Microarray is an efficient tool for circRNA profiling. Brief Bioinform 2018; [Epub ahead of print]. [PubMed]
- Umu SU, Langseth H, Bucher-Johannessen C, et al. A comprehensive profile of circulating RNAs in human serum. RNA Biol 2018;15:242-50. [Crossref] [PubMed]
- Bahn JH, Zhang Q, Li F, et al. The landscape of microRNA, Piwi-interacting RNA, and circular RNA in human saliva. Clin Chem 2015;61:221-30. [Crossref] [PubMed]
- Shao Y, Li J, Lu R, et al. Global circular RNA expression profile of human gastric cancer and its clinical significance. Cancer Med 2017;6:1173-80. [Crossref] [PubMed]
- Bazan HA, Hatfield SA, Brug A, et al. Carotid Plaque Rupture Is Accompanied by an Increase in the Ratio of Serum circR-284 to miR-221 Levels. Circ Cardiovasc Genet 2017;10:e001720 [Crossref] [PubMed]
- Dou Y, Cha DJ, Franklin JL, et al. Circular RNAs are down-regulated in KRAS mutant colon cancer cells and can be transferred to exosomes. Sci Rep 2016;6:37982. [Crossref] [PubMed]
- Lasda E, Parker R. Circular RNAs Co-Precipitate with Extracellular Vesicles: A Possible Mechanism for circRNA Clearance. PLoS One 2016;11:e0148407 [Crossref] [PubMed]
- Li Y, Zheng Q, Bao C, et al. Circular RNA is enriched and stable in exosomes: a promising biomarker for cancer diagnosis. Cell Res 2015;25:981-4. [Crossref] [PubMed]
- Bao X, Zheng S, Mao S, et al. A potential risk factor of essential hypertension in case-control study: Circular RNA hsa_circ_0037911. Biochem Biophys Res Commun 2018;498:789-94. [Crossref] [PubMed]
- Cui X, Niu W, Kong L, et al. hsa_circRNA_103636: potential novel diagnostic and therapeutic biomarker in Major depressive disorder. Biomark Med 2016;10:943-52. [Crossref] [PubMed]
- Huang ZK, Yao FY, Xu JQ, et al. Microarray Expression Profile of Circular RNAs in Peripheral Blood Mononuclear Cells from Active Tuberculosis Patients. Cell Physiol Biochem 2018;45:1230-40. [Crossref] [PubMed]
- Li H, Li K, Lai W, et al. Comprehensive circular RNA profiles in plasma reveals that circular RNAs can be used as novel biomarkers for systemic lupus erythematosus. Clin Chim Acta 2018;480:17-25. [Crossref] [PubMed]
- Ouyang Q, Wu J, Jiang Z, et al. Microarray Expression Profile of Circular RNAs in Peripheral Blood Mononuclear Cells from Rheumatoid Arthritis Patients. Cell Physiol Biochem 2017;42:651-9. [Crossref] [PubMed]
- Pan RY, Liu P, Zhou HT, et al. Circular RNAs promote TRPM3 expression by inhibiting hsa-miR-130a-3p in coronary artery disease patients. Oncotarget 2017;8:60280-90. [Crossref] [PubMed]
- Salgado-Somoza A, Zhang L, Vausort M, et al. The circular RNA MICRA for risk stratification after myocardial infarction. Int J Cardiol Heart Vasc 2017;17:33-6. [Crossref] [PubMed]
- Teng L, Chen Y, Chen H, et al. Circular RNA hsa_circ_0021001 in peripheral blood: a potential novel biomarker in the screening of intracranial aneurysm. Oncotarget 2017;8:107125-33. [Crossref] [PubMed]
- Vausort M, Salgado-Somoza A, Zhang L, et al. Myocardial Infarction-Associated Circular RNA Predicting Left Ventricular Dysfunction. J Am Coll Cardiol 2016;68:1247-8. [Crossref] [PubMed]
- Wu N, Jin L, Cai J. Profiling and bioinformatics analyses reveal differential circular RNA expression in hypertensive patients. Clin Exp Hypertens 2017;39:454-9. [Crossref] [PubMed]
- Zhang J, Xu Y, Xu S, et al. Plasma Circular RNAs, Hsa_circRNA_025016, Predict Postoperative Atrial Fibrillation After Isolated Off‐Pump Coronary Artery Bypass Grafting. J Am Heart Assoc 2018;7:e006642 [Crossref]
- Zhang YG, Yang HL, Long Y, et al. Circular RNA in blood corpuscles combined with plasma protein factor for early prediction of pre-eclampsia. BJOG 2016;123:2113-8. [Crossref] [PubMed]
- Zhao Z, Li X, Gao C, et al. Peripheral blood circular RNA hsa_circ_0124644 can be used as a diagnostic biomarker of coronary artery disease. Sci Rep 2017;7:39918. [Crossref] [PubMed]
- Zheng J, Li Z, Wang T, et al. Microarray Expression Profile of Circular RNAs in Plasma from Primary Biliary Cholangitis Patients. Cell Physiol Biochem 2017;44:1271-81. [Crossref] [PubMed]
- Zhuang ZG, Zhang JA, Luo HL, et al. The circular RNA of peripheral blood mononuclear cells: Hsa_circ_0005836 as a new diagnostic biomarker and therapeutic target of active pulmonary tuberculosis. Mol Immunol 2017;90:264-72. [Crossref] [PubMed]
- Chen S, Li T, Zhao Q, et al. Using circular RNA hsa_circ_0000190 as a new biomarker in the diagnosis of gastric cancer. Clin Chim Acta 2017;466:167-71. [Crossref] [PubMed]
- Li P, Chen S, Chen H, et al. Using circular RNA as a novel type of biomarker in the screening of gastric cancer. Clin Chim Acta. 2015;444:132-6. [Crossref] [PubMed]
- Li WH, Song YC, Zhang H, et al. Decreased Expression of Hsa_circ_00001649 in Gastric Cancer and Its Clinical Significance. Dis Markers 2017;2017:4587698 [Crossref] [PubMed]
- Lu R, Shao Y, Ye G, et al. Low expression of hsa_circ_0006633 in human gastric cancer and its clinical significances. Tumour Biol 2017;39:1010428317704175 [Crossref] [PubMed]
- Sun H, Tang W, Rong D, et al. Hsa_circ_0000520, a potential new circular RNA biomarker, is involved in gastric carcinoma. Cancer Biomark 2018;21:299-306. [Crossref] [PubMed]
- Yang F, Liu DY, Guo JT, et al. Circular RNA circ-LDLRAD3 as a biomarker in diagnosis of pancreatic cancer. World J Gastroenterol 2017;23:8345-54. [Crossref] [PubMed]
- Yin WB, Yan MG, Fang X, et al. Circulating circular RNA hsa_circ_0001785 acts as a diagnostic biomarker for breast cancer detection. Clin Chim Acta 2017; [Epub ahead of print]. [Crossref] [PubMed]
- Zhao Q, Chen S, Li T, et al. Clinical values of circular RNA 0000181 in the screening of gastric cancer. J Clin Lab Anal 2018;32:e22333 [Crossref] [PubMed]
- Zhu X, Wang X, Wei S, et al. hsa_circ_0013958: a circular RNA and potential novel biomarker for lung adenocarcinoma. FEBS J 2017;284:2170-82. [Crossref] [PubMed]
- Broadbent KM, Park D, Wolf AR, et al. A global transcriptional analysis of Plasmodium falciparum malaria reveals a novel family of telomere-associated lncRNAs. Genome Biol 2011;12:R56. [Crossref] [PubMed]
- Boeckel JN, Jae N, Heumuller AW, et al. Identification and Characterization of Hypoxia-Regulated Endothelial Circular RNA. Circ Res 2015;117:884-90. [Crossref] [PubMed]
- Tan WL, Lim BT, Anene-Nzelu CG, et al. A landscape of circular RNA expression in the human heart. Cardiovasc Res 2017;113:298-309. [PubMed]
- Werfel S, Nothjunge S, Schwarzmayr T, et al. Characterization of circular RNAs in human, mouse and rat hearts. J Mol Cell Cardiol 2016;98:103-7. [Crossref] [PubMed]
- Holdt LM, Stahringer A, Sass K, et al. Circular non-coding RNA ANRIL modulates ribosomal RNA maturation and atherosclerosis in humans. Nat Commun 2016;7:12429. [Crossref] [PubMed]
- Khan MA, Reckman YJ, Aufiero S, et al. RBM20 Regulates Circular RNA Production From the Titin Gene. Circ Res 2016;119:996-1003. [PubMed]
- Wu HJ, Zhang CY, Zhang S, et al. Microarray Expression Profile of Circular RNAs in Heart Tissue of Mice with Myocardial Infarction-Induced Heart Failure. Cell Physiol Biochem 2016;39:205-16. [Crossref] [PubMed]
- Ng WL, Marinov GK, Liau ES, et al. Inducible RasGEF1B circular RNA is a positive regulator of ICAM-1 in the TLR4/LPS pathway. RNA Biol 2016;13:861-71. [Crossref] [PubMed]
- Hoefer IE, Steffens S, Ala-Korpela M, et al. Novel methodologies for biomarker discovery in atherosclerosis. Eur Heart J 2015;36:2635-42. [Crossref] [PubMed]
- Cai Y, Yang Y, Chen X, et al. Circulating 'lncRNA OTTHUMT00000387022' from monocytes as a novel biomarker for coronary artery disease. Cardiovasc Res 2016;112:714-24. [Crossref] [PubMed]
- Ren J, Zhang J, Xu N, et al. Signature of circulating microRNAs as potential biomarkers in vulnerable coronary artery disease. PloS One 2013;8:e80738 [Crossref] [PubMed]
- de Gonzalo-Calvo D, Cenarro A, Garlaschelli K, et al. Translating the microRNA signature of microvesicles derived from human coronary artery smooth muscle cells in patients with familial hypercholesterolemia and coronary artery disease. J Mol Cell Cardiol. 2017;106:55-67. [Crossref] [PubMed]
- Graham BB, Chabon J, Gebreab L, et al. Transforming growth factor-beta signaling promotes pulmonary hypertension caused by Schistosoma mansoni. Circulation 2013;128:1354-64. [Crossref] [PubMed]
- Bourcier R, Redon R, Desal H. Genetic investigations on intracranial aneurysm:update and perspectives. J Neuroradiol 2015;42:67-71. [Crossref] [PubMed]
- Devaux Y, Vausort M, McCann GP, et al. MicroRNA-150: a novel marker of left ventricular remodeling after acute myocardial infarction. Circ Cardiovasc Genet 2013;6:290-8. [Crossref] [PubMed]
- Jeck WR, Sharpless NE. Detecting and characterizing circular RNAs. Nat Biotechnol 2014;32:453-61. [Crossref] [PubMed]
- Lyu D, Huang S. The emerging role and clinical implication of human exonic circular RNA. RNA Biol 2017;14:1000-6. [Crossref] [PubMed]
- Sunderland N, Skroblin P, Barwari T, et al. MicroRNA Biomarkers and Platelet Reactivity:The Clot Thickens. Circ Res 2017;120:418-35. [Crossref] [PubMed]
- Ludwig N, Leidinger P, Becker K, et al. Distribution of miRNA expression across human tissues. Nucleic Acids Res 2016;44:3865-77. [Crossref] [PubMed]
Cite this article as: Vea A, Llorente-Cortes V, de Gonzalo-Calvo D. Circular RNAs: a novel tool in cardiovascular biomarker development? Non-coding RNA Investig 2018;2:39.